Under certain conditions, when light falls on a metal surface, the valence electrons of the metal can couple with the electromagnetic fields of the incident radiation, giving rise to a collective movement of the same with the frequency of the fields. These resonances, called plasmons, are particularly important when the metallic structures are reduced to nanometric dimensions which, by allowing the existence of this type of modes, acquire the name of plasmonic nanocavities. Plasmon resonances have a number of very useful properties, such as confinement of radiation at scales smaller than wavelength, generation of torques, and momentum exchange with incident light.
The Study
Now, a team of researchers has studied how to distort the local density of states that exist in a plasmonic nanocavity thanks to the presence of a quantum emitter.
Density of states
Broadly speaking, the quantum description of the physical properties of a system is necessary when its dimensions are reduced to scales little greater than atomic sizes. In this microscopic regime, the energy and the possible states of the system are not continuous, as could be the classic trajectory of a bird flying through the sky. Serve as an example that electrons that travel through a solid cannot do so at any speed and energy, but that there are only certain states for which movement is possible, as well as energy configurations in which electrons cannot move through the medium. . In the context of optics and photonics, the density of states refers to the different energy configurations that an excitation of the system can take, which we commonly call a photon. Typically, there will be certain energy values that allow the existence of photons in the system and others in which the presence of a photon is impossible. In the first case, since we are dealing with a system that has accessible energy states, we will say that the density of states has a positive value, which will be greater the more accessible states exist at that energy. In the second, the density of states will be zero. The joint resonances of the electrons and the electromagnetic fields of the plasmon cavities take this discrete form: there will only exist certain permitted states of energy, which will be able to exchange said energy with their environment.
Quantum emitter
As we have already indicated previously, when dealing with systems made up of few atoms, the states of the system are discrete. If we consider one of these systems in an excited state, different from the ground state of lowest energy, different mechanisms can promote its relaxation towards the state of minimum energy, while the system emits radiation with the difference in energy between the two states. This type of system is what we call a quantum emitter, capable of relaxing by emitting a single photon. The most paradigmatic example of a quantum emitter is the atom, but there can be more complex systems, such as molecules, ions or quantum dots that can also have this emission property.
During the last decade, the combination of quantum emitters and plasmonic resonances has allowed access not only to the study of different regimes of the interaction between plasmons and emitters. Plasmon cavities have emerged as one of the key technologies for exploring and exploiting the properties of light-matter interactions at the nanoscale, leading to a plethora of research and applications in areas such as
optomechanics , biosensing or spectroscopy. All of them make use of the remarkable intensity of plasmon-emitter interactions that originate in metallic structures at the nanoscale.
The development of different nanofabrication techniques is progressively overcoming the difficulties inherent in controlling both the exact number of quantum emitters and their exact position and orientation within a nanocavity. In this line, a considerable part of the current investigation of the plasmon-emitter interaction in nanometric systems is oriented to achieve a regime of the interaction called strong coupling, where the quantum emitter and the plasmonic modes form hybrid states of light and matter, called polaritons, or more specifically in the context of plasmonic nanocavities, plexcitons.
In this theoretical study, two emitters are considered in a plasmon cavity, as seen in the figure, whose interaction with each of the emitters separately is known. The first emitter plays the role of detecting the density of states resulting from the cavity, modified by the presence of the second emitter.
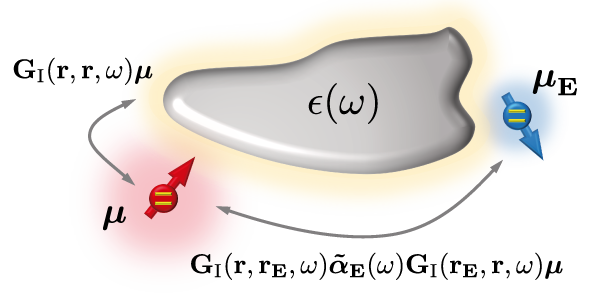
div>
The group of researchers has developed a theory that describes the action of the second emitter from the interactions of the stripped cavity, without the presence of the second emitter. The results have been obtained for two cavities, for which they have verified the validity of the theory through numerical simulations and, finally, have provided an interpretation of their results in terms of recent theoretical results on the quantization of plasmonic modes. The results reflect that the presence of the second emitter can significantly increase or reduce the density of states of the cavity.
This new work offers a new perspective on nanoscale quantum emitter interactions, with special relevance in the context of transport phenomena of
excitons , in quantum optical metamaterials, as well as in the design and engineering of picocavities, where subnanometric characteristics greatly alter their local density of states.
The work is a collaboration between the Department of Theoretical Physics of Condensed Matter and the Institute of Physics of Condensed Matter (IFIMAC), both attached to the Autonomous University of Madrid, the Department of Physics and Astronomy of the University of New Mexico and the Institute of Optics of the CSIC.
Access to the full article